quantum interference manipulation
- Mohammed KM
- Dec 7, 2024
- 10 min read
Updated: Dec 8, 2024
Quantum physics is a relatively new development in science which entails a radically new understanding of the world along with the potential to unlock very new technological innovations. Laws of classical physics i.e. Newtons laws of motion beautifully (and intuitively) explain the physical phenomenon of objects we observe in our day-to-day life at a macroscopic level. For example, we can imagine two wide slits (i.e. wide enough to allow a tennis ball to pass) placed before a wall and two tennis balls coated with wet paint are shot through each of the slits. Unsurprisingly, the two balls travel in straight lines passing through their respective slits and hit the wall leaving two ball-sized paint marks on it. The trajectories of the tennis balls are intuitively understandable and can be easily determined by Newtons laws of motion to determine which part of the wall gets hit by the balls. If we shoot only tennis ball through the slit, we observe only one ball-sized mark on the wall. The reason I mention this hypothetical experiment is to contrast this with a similar experiment that was actually conducted (i.e. the famous double slit experiment) which contributed to giving rise to a new and slightly controversial branch of physics which is quantum physics. In the double slit experiment, two very narrow slits (which can allow an electron or light photon to pass through) were constructed and placed before a photo-sensitive screen which is analogous to the wall in the tennis ball experiment. Before we dive deeper into the experiment, let us try to understand a bit about an important property of light, after all this property is what ultimately led to the birth of quantum physics. Even though light may appear like a continuous stream of energy, in actuality it is a stream of discrete or rather quantized (hence the ‘quantum’) packets of energy which has been proved by various experiments. An interesting thought experiment can help understand the quantized nature of light a bit better: imagine a frog (its eyes are highly light sensitive in comparison to humans) that is placed far far away from a light source such that what appears to be single ray of light is all that enters the frog's eye but what the frog’s eye experiences are actually discontinuous flickers of light where each flicker corresponds to one quantized packet or photon of light entering the frog’s eye. Now coming back to the double slit experiment, two light beams are allowed to pass through the two slits, rather than observing two slit-sized light streaks on the screen (analogous to tennis ball-sized marks on the wall), we oddly observe a pattern of many alternate light and shadow streaks called an interference pattern. The same interference pattern was observed when we repeated the experiment with electron beams instead of light beams which started raising a lot of questions as light could have been considered as an electromagnetic wave (even though it has particle-like properties owing to photons) to potentially explain why we observe the interference pattern, but an electron was always known to be a particle. In addition to this, a modified version of the double slit experiment started to raise even more alarming questions i.e. when the experiment was constructed in a certain way such that only one photon or electron was fired at the double slit at a time, we still observed the same interference pattern as we would have observed if two electrons or photons were fired at a time with one particle passing through each slit. In the hypothetical tennis ball experiment, one ball fired at a time would produce a singular spot on the wall rather than a double spot that is observed when two balls are fired at the same time. Newtonian physics which appeared complete at the time and could magically explain a wide range of physical phenomenon utterly failed to explain the occurrence of the interference pattern observed in the case of minute particles like photons or electrons. This led to the unfortunate conclusion that we had an incomplete understanding of what reality is and we direly needed a new understanding of what reality actually entails. This led to the birth of quantum physics and some very interesting theories on explaining the new reality like the quantum realm and parallel universes. Wave-particle duality theory (i.e. the property of matter to behave like both a wave and particle where wave-like properties are more prominent for matter of smaller dimensions) and superposition served as good explanations for the unusual interference pattern observed for electrons and photons in the double slit experiment where wave-like properties of the electrons or photons is exhibited at the slits and interaction at the slits causes superposition i.e. addition of these waves in order to produce a unique interference pattern rather than a plain set of two slit-sized streaks on the screen. Quantum interference serves as a very interesting explanation to understand the more unusual observation of an interference pattern being observed when a single photon or electron was fired through the slits. If one of the slits had been covered and then we fired a beam of photons or electrons through the slits, the pattern observed is starkly different from the case in which both slits are open, which gives rise to the conclusion that the interference pattern formed is a result of interaction between photons or electrons passing through both the slits. However, this still does not explain why an interference pattern is observed for a single photon or electron fired through the slits similar to the case when multiple photons are fired because if a single particle is fired, what does it interact with to produce the observed interference pattern? Does the single particle split into two so that it can pass through both the slits to be able to interact and generate the observed interference pattern? In the single particle case, the singular photon or electron has to interact with another entity that possesses the exact same property of a photon or an electron to logically explain that an interaction takes place, giving rise to the formation of the observed interference pattern. This is where the interesting proposition of the quantum realm comes into the picture. British physicist David Deutsch and a strong proponent of the existence of parallel universes, theorizes that the singular photon or electron (which is observable and measurable) interacts with a ‘shadow’ photon or electron (which is basically an unobservable and unmeasurable counterpart of the observable particle in another identical parallel universe) through a special phenomenon called quantum interference where our tangible universe is more observably appearing to interact with another intangible parallel universe, in order to produce the same interference pattern that would be observed if two observable and measurable photons or electrons interacted with each other. Quantum superposition is an interesting theory which in essence states that matter can simultaneously exist in multiple states at the same time until the time it is observed during which it collapses into any one of the multiple states. If we try to translate quantum superposition into the parallel universe theory, it will look something like this: the same particle can exist in different states in identical parallel universes with the possibility of interacting with each other until an observation is made. Let us take an example to understand this better, lets us shine a single photon at a semi-silvered mirror where there is 50% chance for it to either pass through or get reflected. Until we have made an observation using a measuring instrument, we have no idea if the photon has passed through or gotten reflected. In the parallel universe theory, the photon would have passed through in 50% of identical parallel universes i.e. state 1 and gotten reflected in the remaining 50% of identical parallel universes i.e. state 2. So, until the observation is made, the final state of the photon is like a type of coupling of multiple photons (of effectively two different states i.e. state 1 or state 2) in identical parallel universes in which random inter-universe interactions between the photon of our universe and the shadow photons i.e. counterparts of other universes is possible up until the final observation is made, where the state of the photon ultimately collapses into one of the possibilities in the vast collection of universes i.e. either one of the many universes where the photon has passed through the semi-silvered mirror or one the equivalently many universes where the photon gets reflected. This example in a way states that every simple observation made on a particle is probably an unapparent complex result of quantum mechanical interactions of the particle and its counterparts from identical parallel universes. Quantum mechanical interactions are more visible for matter of minute dimensions like an electron or photons and hence these types of particles have been aptly termed as quantum mechanical particles. This potential new understanding of reality that was incepted due to unusual observations from the double slit experiment sounds radical but is an important steppingstone to an inevitable fact that our currently understanding of what reality truly is in actuality grossly limited.
Now that we might have gotten some level of understanding of the special phenomenon of quantum interference, it is now time to dive into expanding on the title of this article which is manipulating the quantum interference phenomenon. Quantum computing is a promising technological development where we are able to positively manipulate the phenomenon of quantum interference to our advantage to devise a new computational structure which can innovatively (and tractably) perform certain operations which is impossible for a standardly used classical computer to perform in a realistic time frame. Classical computers operate on the concept of binary information called bits (1 or 0) which is basically one of two electrical states i.e. 1 when there is an electric current in the matter or 0 when there is no electric current in the matter. Transistors which are semiconductors have the unique property to be in a binary electrical state thereby allowing it to store and manipulate binary information. The beauty of the binary information system which is the bedrock of computer science lies in the fact that any type of information (from texts, photos, videos, audio, computational instructions etc.) all can be ultimately condensed into a unique sequence of 1s and 0s which is ultimately sequence of dynamic electrical signals rendered on transistor circuits. A classical bit element contained in an elementary transistor can store only one information state at a time i.e. either 1 or 0 but not both. Quantum computers were incepted to bypass this particular limitation of classical computers and thereby widen the possible computational capacity of existing computers. Quantum computers make use of quantum mechanical objects like electrons or photons to store and manipulate information in the form of quantum bit or qubits. Analogous to classical bits that use the presence of electrical current to represent the binary information states, quantum bits make use measurable properties of the electron (i.e. spin value) or photon (i.e. phase) in order to represent the information states. The spin value and phase are ultimately measures of how the electron wave or light wave is spatially positioned with respect to its axis of propagation during its state of motion. So, an electron can be in one particular spin state which could correspond to the 1 in classical computer transistors and an opposite spin state which could correspond to the 0 in classical computer transistors. So, qubits also store information in 1s and 0s like classical bits but with an added advantage: qubits utilize the principle of quantum superposition where until measured, qubits can store multiple information states at the same time in comparison to classical bits which store only one information state at a time. For example, if we consider a 2-bit information system, we have 4 possible values (00, 01, 10, 11) and a classical bit can store only one of these 4 states at a time but a quantum bit can theoretically store all the 4 states (with a specific probability to enter into each state prior to measurement) at the same time until the time it is actually measured or observed after which it collapses into one of the 4 states. So, if we try to perform an operation on information stored in a classical bit through a function say f(x), only one function out of 4 { f(00), f(01), f(10), f(11) } can be executed at a time but if the same function is applied on a qubit, all 4 of the functions can be executed simultaneously after which it ultimately collapse into one of the 4 functions when the final observation is made. If quantum bits ultimately collapse into one of the 4 states, how is it really different from a classical bit and how do we make use of the supposed advantage of quantum bits? The trick lies in manipulating the probabilities of which each quantum state could collapse into when the measurement is finally made. Let us try to understand this a bit better: certain computations have a mathematical purpose to reach a desired type of output and we can try to manipulate the information stored in quantum bits or qubits as per our purpose through use of quantum algorithms built on top of quantum gates (analogous to classical computer algorithms built on top of logic gates like AND, OR etc. ) such that the output of these algorithms is again a superposition of different possible information states with different probabilities of being the final output when actually observed but manipulated in such a way that one of the possible states which suits our mathematical purpose has now a disproportionately higher probability of being the final output when actually observed. So, the benefit of quantum computers is being able to parallelly perform mathematical operations on multiple information states stored in qubits at the same time and then manipulating the outputs of these operations such that the unfavorable outputs can be probabilistically discarded and the favorable output has a high probability of being the final observed state. Shor’s algorithm is a potential application of quantum computing which can (if practically implementable) be able to break the encryption for secured data generally transferred between different computer systems across the world i.e. the internet. Breaking the encryption for secured data on the internet requires being able to factorize a very large number (i.e. public key which possessed by everyone) to obtain its prime factors (one of which will be the private key which is the key security element that is possessed only by the owner) which is not realistically possible with classical computers due to computational limitations but theoretically possible for quantum computers through a very interesting process that uses Shor’s algorithm. Using the principle of quantum interference, a quantum computer is effectively able to distribute a set of additional computational tasks parallelly across multiple parallel universes with each universe having a certain probability for its computed output to be the final observed output whereas a classical computer needs to accumulate additional computational resources within the same universe in order perform the additional computational tasks. However, a key challenge for quantum computer arises due to a property of quantum mechanical objects called decoherence. While quantum mechanical objects can be in a state of superposition of multiple quantum states until the observation is made, the object can prematurely collapse into one of the states (in a way or time that we don’t prefer) due to uncontrollable interactions with the external environment, which causes decoherence. This ends up affecting the information stored and calculations being performed in the quantum computer. This limitation can be potentially mitigated by the use of superconductors and extreme cooling which can reduce the uncontrollable interactions with the external environment but superconductors along with quantum computers are still in their nascent stages of development as of now and there is still a long way to go.
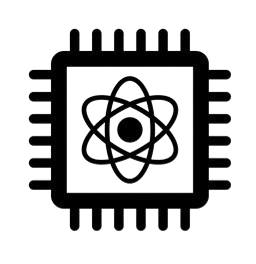